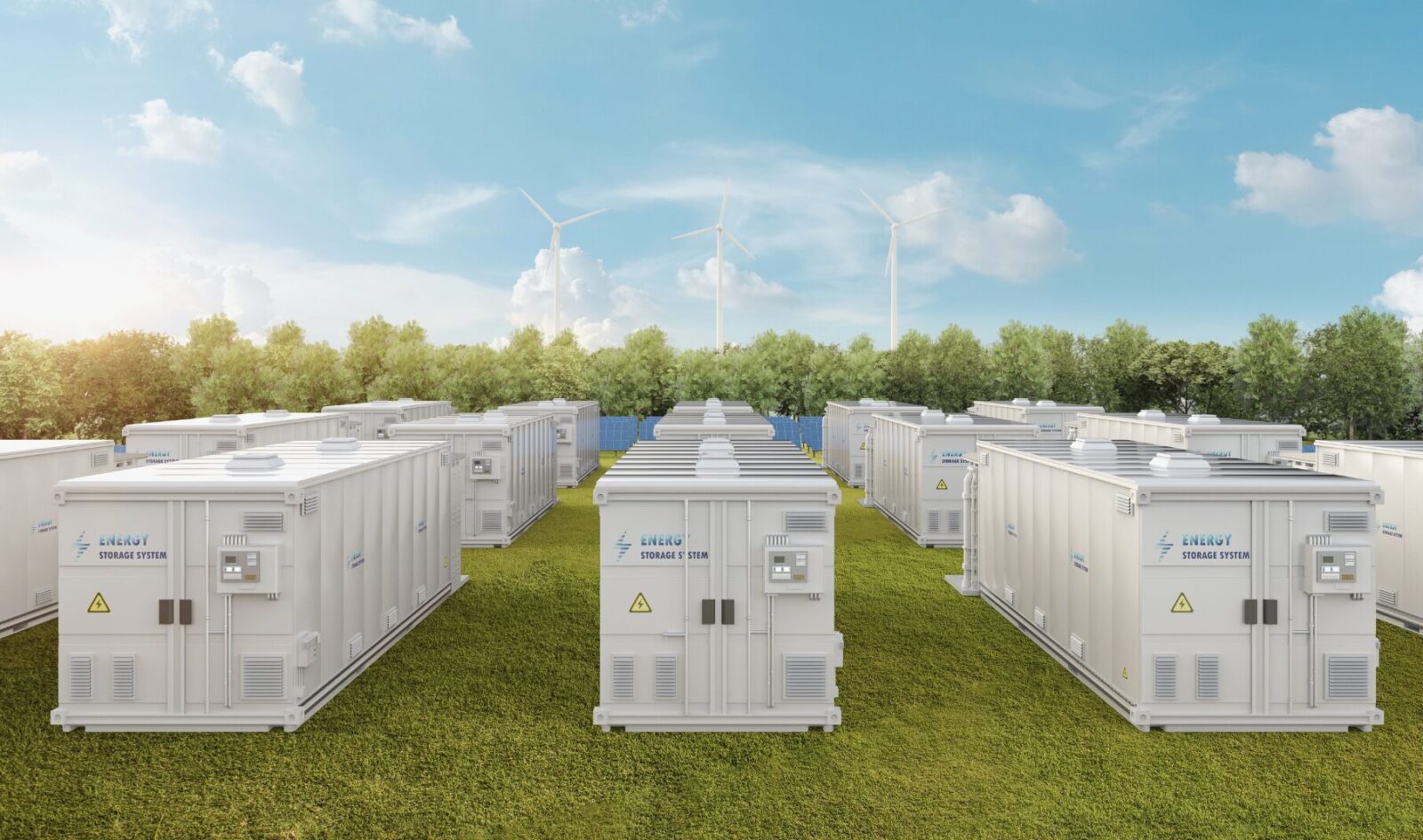
With 60% of global greenhouse gas emissions coming from energy, there’s a universal need to make our power system as clean and cost-effective as possible. Renewable energy sources like solar and wind are excellent options, but they’re intermitten by nature, meaning they’re effective only when the sun is shining and the wind blowing.
To overcome this challenge, grid-scale energy storage systems are being connected to the power grid to store excess electricity at times when it’s plentiful and then release it when the grid is under periods of especially high demand. Deployments of these systems have increased dramatically over the last several years, to 17 Gigawatts at the end of 2023—enough to power approximately thirteen million homes. The role of energy storage in accelerating our transition to renewables is why Alsym Energy is developing a high-performance, low-cost and non-flammable battery focusing on grid-scale battery storage.
What Is Grid-Scale Battery Storage?
When asked to define grid-scale energy storage, it’s important to start by explaining what “grid-scale” means. Grid-scale generally indicates the size and capacity of energy storage and generation facilities, as well as how the battery is used. The size of a battery storage facility is its standard physical dimensions, and the capacity is the amount of electricity the facility can put out and store, measured in kilowatt hours (kWh), megawatt hours (MWh), gigawatt hours (GWh), and at some point in the future terawatt hours (TWh).
Grid-scale is different in terms of battery size and use cases than residential scale or commercial and industrial sale. Here is a breakdown of the differences between the three main levels of energy storage systems:
- Residential systems: Homeowners can install solar panels on their roofs and pair their onsite generation with a personal battery, typically sited in their garage, basement, or another discrete location. Common ranges of residential battery systems are from 3kWh to 15kWh, and the most common use cases include load shifting and backup power during outages.
- Commercial systems: These systems are larger, powering businesses, hospitals, and schools. Some larger battery systems are making their way into manufacturing plants which have very high power demands. They store up to several megawatts of power and are found usually alongside parking lots. These are often part of private microgrids that may also include chemical generation (diesel generators or fuel cells) or renewable generation (solar and wind), and tie in to the public grid.
- Grid-scale systems: These are the biggest batteries, often over a hundred megawatts in capacity. Grid-scale systems are typically managed by utilities or independent power producers (IPPs) and can supply entire regions with electricity. The most popular use cases for grid-scale energy storage systems are peak shaving, frequency regulation, and arbitrage, although that list is expanding into new applications.
There are behind-the-meter (BTM) and front-of-the-meter (FTM) energy storage systems, and it’s important to know the difference. BTM systems generate and use energy directly on-site without passing through an electric meter. BTM systems can still be connected to the electric grid but manage the renewable and storage systems independently from it. They include home solar panels with on-site energy storage, and microgrids. FTM interacts with the central power grid, including generation facilities like coal, gas, wind, solar, and geothermal plants, utility-sized energy storage facilities, and transmission and distribution lines.
Today’s systems using lithium-ion batteries are different from those pumped hydro, compressed air, or gravity because they are fast, providing power almost instantly when needed. They are also scalable if there’s a need for expansion. Batteries are also portable, unlike other storage systems that need to be on mountaintops or in underground tunnels.
Grid-Scale Batteries
There are several different types of grid-scale batteries, and each has their own applications and specifications, including:
- Lithium-ion battery energy storage systems are the most common electrochemical battery and can store large amounts of energy. Examples of products on the market include the Tesla Megapack and Fluence Gridstack.
- Flow batteries for grid-scale energy storage collect energy in liquid electrolytes, have a long cycle life, and are scalable. Popular examples are the vanadium redox battery (VRB) and iron-flow battery.
- Sodium-sulfur (NaS) batteries are known for energy efficiency and the ability to withstand many charging cycles. The NaS battery from NGK Insulators is one example.
- Advanced lead-acid batteries are improved versions of traditional batteries and offer better performance. They include technologies like absorbent glass mat (AGM) and gel batteries.
A battery energy storage system comes together through a number of industry players. The building block of energy storage systems are battery cells, produced by major global companies like CATL, BYD, and LG Energy Solution. These battery cells are then integrated into systems of varying size by integrators such as Fluence and Powin and outfitted with a battery management system (BMS), thermal regulation equipment, and other operation-critical components. From here, a developer or installer designs and deploys the energy storage system, including the battery containers, control systems, and grid connections including transformers and inverters, which are typically manufactured by companies unrelated to the battery itself. The developers integrate components into functional grid-scale energy storage solutions.
Efficiency and Lifespan
Grid-scale batteries have a round-trip efficiency (RTE) measurement, which shows the energy lost during storage and retrieval, usually 70–90%. Lithium-ion batteries reach an industry-high RTE of 90%+, lead-acid measures about 70%, flow batteries are around 50–75%, and metal-air designs can be as low as 40%. A crucial component to the cost-effectiveness of a battery is mitigating the energy wasted during charge and discharge.
The lifespan of a grid-scale battery depends on its chemistry, how long the battery has been used, and how often it’s charged and discharged. Applications of lithium-ion batteries in grid-scale energy storage systems last about 10–15 years. Lead-acid is between 5–10 years. Another factor is where the batteries are stored, as batteries kept in higher or very low temperatures can experience a shorter lifespan.
Benefits of Grid-Scale Battery Storage
Energy systems that use grid-scale battery storage are more reliable, efficient, and environmentally friendly. A top benefit is the ability to stabilize the grid during fluctuations from renewable sources. They store energy during low demand, like the sunny afternoon or a windy night, and then release that energy during peak demand times. A grid-scale battery also regulates frequency by responding quickly to changes in generation and demand, which leads to cost savings.
Another advantage is blending renewable energy sources into the grid. Weather can be unpredictable and therefore so is the power generated by it. A grid-scale battery stores energy when there’s no wind and the sun isn’t out. Batteries maximize the use of renewable energy and help move away from fossil fuels.
Grid-scale batteries can also provide a crucial backup system during outages or emergencies. They provide power to essential services like communication networks, hospitals, and emergency services, making sure they are always operational. Grid-scale battery storage balances supply and demand, improves dependability, lowers costs, and ultimately offers a sustainable energy solution.
Barriers to Grid Energy Storage
There are some obstacles standing in the way of increased adoption of grid-scale energy storage, including:
- Material availability: Both the EV and the grid-scale energy sectors rely on the same materials, like lithium, cobalt, and nickel. Further, only a handful of companies control the lithium-ion battery supply for both segments.
- Thermal runaway and toxicity: While lithium-ion batteries are very good at storing and discharging energy, they pose extremely dangerous hazards such as the potential to go into thermal runaway and to release toxic fumes during fire incidents. As a result, strict safety protocols and regulation challenges have arisen.
- Duration challenges: In general different technologies can only discharge cost-effectively for a specific time window. For lithium-ion batteries it’s 6 hours or less. For iron-air batteries it’s 100 hours or more. Discharge duration needs change as the grid evolves and battery technology will need to evolve as well.
The Future of Grid Energy Storage
Startups are leading the charge with non-lithium battery chemistries to overcome the barriers above and promise a safer, more affordable, greener future. Many integrators are “chemistry agnostic,” meaning they’re prepared for the next wave of battery technologies.
Alsym Energy has developed a cost-effective, high-performance, rechargeable battery technology that doesn’t use cobalt or lithium. It leverages readily available materials that are non-flammable and non-toxic. Alternative battery chemistries like Alsym’s are designed with affordability in mind to ensure everyone has access to clean energy.
There’s an urgent need for low-cost, clean energy solutions to reach net-zero greenhouse gas emissions by 2050 and mitigate the far-reaching impacts of climate change. The intermittent nature of renewable energy sources requires a backup plan. Grid-scale energy storage is vital for the future of renewable energy and to meet the changing demands of the grid. Alsym’s innovators are on the case by working to develop a novel battery technology for a sustainable tomorrow.